5 Major Challenges in Space Navigation
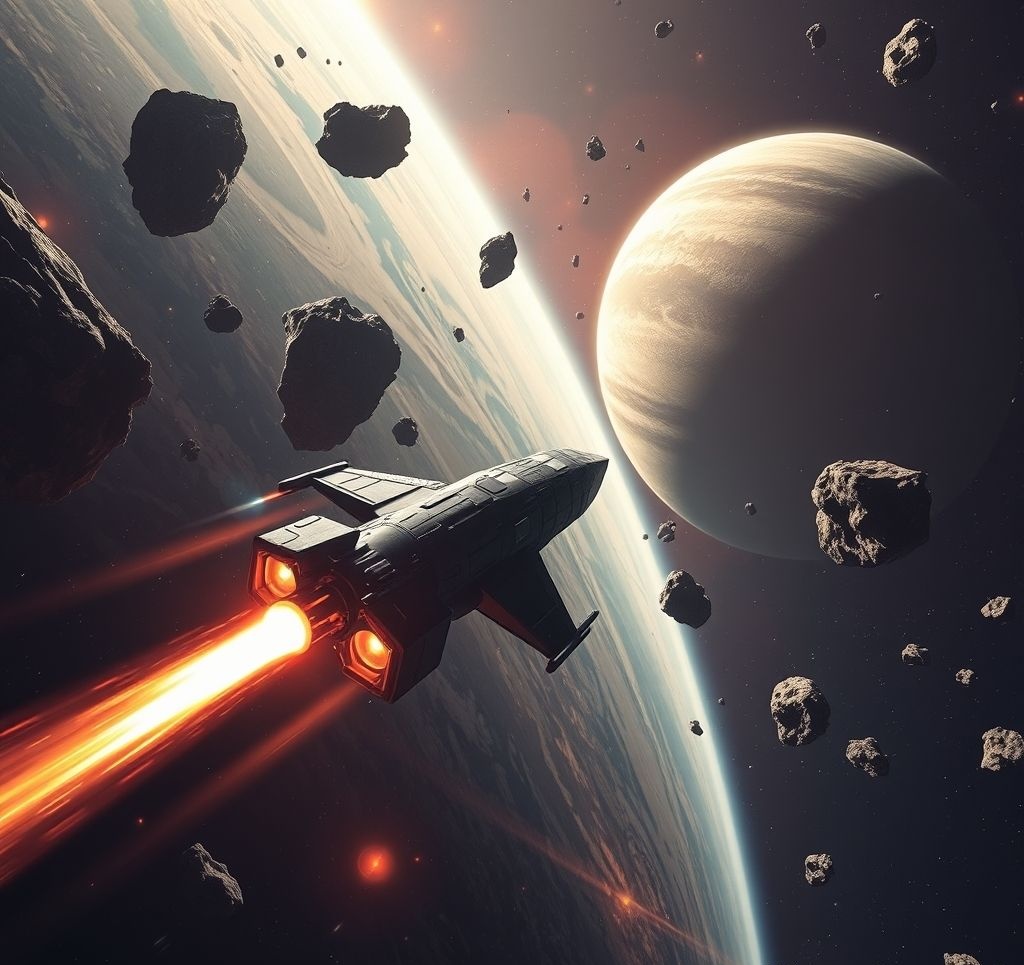
When we think of navigation challenges, most of us imagine finding our way through a dense forest or negotiating a complex urban environment. But these pale in comparison to the extraordinary challenges faced by engineers and mission planners responsible for navigating spacecraft through the vacuum of space. In this article, we'll explore the five most significant challenges in space navigation and the ingenious solutions developed to overcome them.
In This Article:
Challenge 1: The Light-Time Delay Problem
Perhaps the most fundamental challenge in deep space navigation is that radio signals—which travel at the speed of light—take significant time to traverse the vast distances between Earth and spacecraft.
Light-Time Delays to Various Destinations:
- Moon: ~1.3 seconds (one-way)
- Mars: 3-22 minutes (one-way, depending on orbital positions)
- Jupiter: 35-52 minutes (one-way)
- Pluto: ~5.5 hours (one-way)
- Voyager 1: ~21 hours (one-way) and increasing
These delays make real-time control from Earth impossible for most deep space missions. When NASA's Perseverance rover landed on Mars in 2021, the entire entry, descent, and landing sequence had to be pre-programmed and executed autonomously. By the time mission control received the signal that the landing had begun, Perseverance was already safely on the surface or had crashed—there was no way to intervene.
Current Solutions:
- Event-Driven Sequences: Detailed command sequences that respond to specific events rather than precise timing
- Predictive Commanding: Commands based on where objects will be, not where they were observed
- Increasing Spacecraft Autonomy: Allowing spacecraft to make more decisions without Earth input
"We don't fly spacecraft in real-time. We fly them in what I call 'anticipatory time'—we have to anticipate what the spacecraft will encounter and program responses to events that haven't happened yet." — Dr. Nagin Cox, NASA/JPL Mission Operations Engineer
Challenge 2: Navigation Uncertainty
Even with our most sophisticated tracking techniques, there's always some uncertainty in our knowledge of exactly where a spacecraft is and how fast it's moving. These uncertainties grow with distance from Earth and can have serious consequences for mission success.
Several factors contribute to navigation uncertainty:
- Measurement Limitations: Even using the Deep Space Network's giant antennas, range and velocity measurements have inherent limitations in precision
- Gravitational Perturbations: Small gravitational forces from distant objects can gradually affect trajectories
- Non-Gravitational Forces: Solar radiation pressure, outgassing, and tiny thruster imbalances create small but cumulative effects
- Computational Approximations: Mathematical models used for trajectory calculations are necessarily simplified
The New Horizons Pluto Flyby Challenge
When NASA's New Horizons spacecraft flew by Pluto in 2015, it was traveling at 14 km/s (31,000 mph) and had to pass through a target zone just 100 by 150 kilometers wide from a distance of 4.8 billion kilometers away. This was equivalent to hitting a golf ball from Los Angeles to New York and getting a hole-in-one.
To achieve this precision, the team used a combination of radio tracking from Earth, optical navigation (using the spacecraft's cameras to observe Pluto against star backgrounds), and multiple course correction maneuvers.
Current Solutions:
- Regular Trajectory Correction Maneuvers: Small burns that adjust course based on updated position measurements
- Optical Navigation: Using cameras to determine position relative to target bodies and stars
- Statistical Orbit Determination: Sophisticated algorithms that combine multiple measurement types to reduce uncertainty
- Safety Margins: Planning trajectories with sufficient margins to account for position uncertainty
Challenge 3: Radiation Effects on Navigation Systems
Space is filled with radiation that can wreak havoc on the sensitive electronics required for precise navigation. This radiation comes from multiple sources:
- Solar Radiation: Particularly during solar flares and coronal mass ejections
- Cosmic Rays: High-energy particles from outside our solar system
- Trapped Radiation: Particles held in planetary magnetic fields (like Earth's Van Allen belts)
- Radiation from RTGs: Some spacecraft use Radioisotope Thermoelectric Generators for power, which produce radiation
These radiation sources can cause several types of problems for navigation systems:
- Single Event Upsets (SEUs): Bit flips in computer memory that can corrupt navigation data
- Single Event Latchups (SELs): Potentially damaging short circuits in electronics
- Total Ionizing Dose (TID) Effects: Cumulative damage that degrades electronic performance over time
- Star Tracker Interference: Radiation "noise" that can confuse optical navigation systems
Current Solutions:
- Radiation-Hardened Electronics: Specially designed components that resist radiation damage
- Error Detection and Correction: Software that can identify and fix corrupted data
- Redundant Systems: Multiple copies of critical navigation hardware
- Shielding: Physical barriers that block some forms of radiation
- Safe Mode Programming: Automated responses that protect spacecraft when navigation systems are compromised
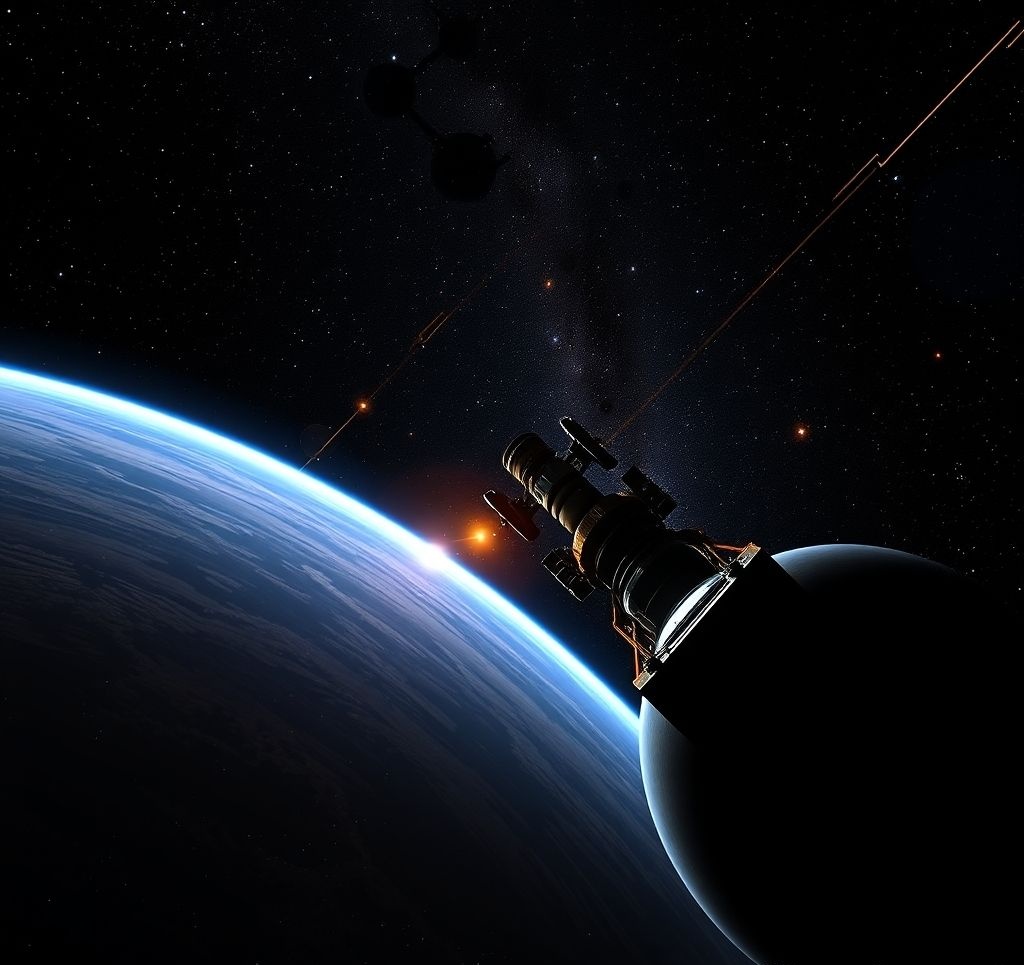
Radiation effects on spacecraft electronics can cause navigation errors if not properly mitigated.
Challenge 4: Limited Resources and Autonomy
Space missions operate under severe constraints that directly impact navigation capabilities:
- Power Limitations: Especially for missions beyond Mars where solar power is insufficient
- Computational Constraints: Space-qualified computers lag behind consumer technology
- Communication Bandwidth: Limited data rates restrict how much navigation information can be transmitted
- Propellant Constraints: Once launched, spacecraft have finite ability to change their trajectory
These limitations are particularly challenging for missions requiring high levels of autonomy, such as comet and asteroid rendezvous, planetary landings, and sample return missions.
OSIRIS-REx Autonomous Navigation Challenge
NASA's OSIRIS-REx mission to asteroid Bennu faced the daunting task of navigating to a specific sampling site on a small, irregularly shaped asteroid. The spacecraft had to autonomously avoid hazards during its final descent while operating with limited onboard computing power and a 20-minute round-trip communication delay.
The solution was the Natural Feature Tracking (NFT) system, which compared real-time camera images with an onboard map of Bennu's surface to determine position and navigate precisely to the sampling location. This autonomous system enabled the spacecraft to collect a sample from a target area the size of a few parking spaces on an asteroid 200 million miles from Earth.
Current Solutions:
- Efficient Algorithms: Navigation software optimized for limited computational resources
- Selective Data Transmission: Prioritizing critical navigation data in limited bandwidth
- Low-Energy Trajectories: Routes that minimize propellant requirements
- Sliding Autonomy: Systems that can operate at different levels of independence based on circumstances
- Hardware/Software Co-design: Developing specialized hardware that efficiently runs navigation algorithms
Challenge 5: Navigating Unknown Environments
Perhaps the most exciting challenge in space navigation is dealing with the unknown. When spacecraft visit destinations for the first time, they must navigate environments with significant uncertainties:
- Uncertain Topography: Detailed surface features may be unknown until arrival
- Unknown Gravitational Fields: Small bodies like asteroids have complex, poorly mapped gravity
- Unpredictable Environments: Dust, outgassing, and other phenomena can affect navigation
- Limited Prior Mapping: Navigation references may be based on low-resolution remote observations
These challenges are particularly acute for landing missions and for navigation around small bodies like comets and asteroids.
Current Solutions:
- Terrain Relative Navigation: Using cameras to match surface features with stored maps in real-time
- Lidar and Radar Mapping: Active sensors that can create detailed topographic models during approach
- Conservative Approach Trajectories: Planning cautious initial approaches to unknown environments
- Progressive Reconnaissance: Gradual mapping of environments before attempting critical maneuvers
- Hazard Detection and Avoidance: Autonomous systems that identify and avoid dangerous areas
"The reality of space exploration is that we're frequently asked to navigate precisely to places we've never been before, using incomplete information and autonomous systems. It's like asking someone to parallel park a car in a space they've only seen in a blurry satellite photo—from a thousand miles away." — Miguel San Martin, Chief Engineer for Guidance and Control, Mars 2020 Mission
Future Solutions and Technologies
As we push the boundaries of space exploration, numerous technologies are being developed to address these navigation challenges:
Advanced Autonomy
Future spacecraft will feature increasingly sophisticated autonomous navigation capabilities:
- Machine Learning Systems: Algorithms that can adapt to unexpected conditions
- Cognitive Architectures: Systems that reason about navigation problems more like humans
- Collaborative Autonomy: Multiple spacecraft that share navigation information
Interplanetary Navigation Infrastructure
Just as GPS revolutionized Earth navigation, dedicated navigation infrastructure will transform deep space operations:
- Deep Space Navigation Satellites: Dedicated navigation beacons around planets and moons
- Pulsar Navigation: Using the regular signals from pulsars as a natural galactic positioning system
- Interplanetary Internet: Network infrastructure allowing more robust navigation data sharing
Quantum Technologies
Quantum physics offers revolutionary potential for navigation:
- Quantum Sensors: Ultra-precise accelerometers and gyroscopes for inertial navigation
- Optical Atomic Clocks: Time references orders of magnitude more stable than current systems
- Quantum Communication: Potentially enabling more secure and efficient navigation data transmission
The Continuing Evolution of Space Navigation
Space navigation has come a long way since the days of the Space Race when engineers calculated trajectories using slide rules and mainframe computers. Today's deep space missions rely on sophisticated autonomous systems, precision tracking networks, and advanced mathematics to find their way through the cosmos.
As we look to the future, with ambitious goals like Mars sample return, asteroid mining, and human missions to deep space, navigation technology will continue to evolve. The challenges remain immense, but the solutions being developed today will enable the next generation of space exploration, extending humanity's reach further into the solar system and beyond.
Comments
Leave a Comment
Be the first to comment on this article!